2. Introduction¶
This document is the record of the graduate research project conducted by Brent Huisman from October 2011 to August 2012 for the P&AP master program of the University of Amsterdam, at the Nikhef institute, in the Detector R&D group. About half a year before the start of my work, a project was started in the Detector R&D group on a proton imaging system based on the Medipix family of detector chips, intended for medical imaging. My research task was the continuation and enhancement of this proton beam monitor setup. Together with the people who started the project, the proof of principle was delivered by testing the setup at the KVI accelerator in Groningen. The test was successful, but many points for improvement were discovered. Properly charting these improvements and making a start with the implementation was my main occupation during the project.
At first glance this project may seem far removed from the topic of the P&AP master program. This field and therefore the master program is concerned with the characterization of the fundamental building blocks of matter, space and time, with which it is hoped we can develop a greater understanding of the world around us. Technology and knowledge acquired for fundamental research of this type can, and is, applied in a plethora of other fields however. One such field does so with huge success, and that field is medicine. Inspired by a lecture on proton therapy by Ugo Amaldi at the Cern Summer School of 2011, I chose the option provided by the Nikhef Detector R&D group to work on a medical proton imager. Next to constructing images for diagnostic use, this system can be used to obtain an accurate stopping power distribution necessary for treatment which is now commonly obtained via a converted X-ray CT image. Part of any radiological treatment is the construction of an image of the tissue distribution, which is used to calculate the best treatment plan. Particle beams and X-rays probe different properties of matter, so a conversion from one radiodensity (X-ray) to another (protons) has an inherent error that translates to an extra inaccuracy. To remove this conversion would be an improvement.
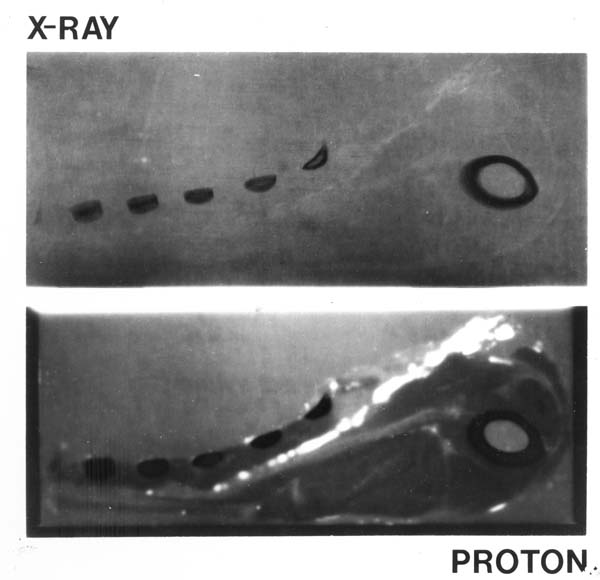
Figure 1: A comparison of an X-ray image (top) and a proton image (bottom) of a lamb chop, the first published proton image by Koehler (1968).
Proton beam imaging is more sensitive to certain differences in tissue than X-ray imaging, and delivers less radiation dose while doing so (Hanson et al., 1981, Kraft, None, Schulzertner et al., 2004, Depauw and Seco, 2011, Amaldi and Kraft, 2005, Kraft, 2000, Zygmanski et al., 2000, Schardt and Elsässer, 2010). While medical proton beam imaging is not a new idea (figure 1 (Wilson, 1946, Koehler, 1968, Hanson et al., 1981)), a novel approach was employed in this Nikhef project. Within the Detector R&D group much research is conducted with the MediPix detector chip family, which is a programmable two dimensional charge detector. This project uses such chips as part of the proton beam monitor, whereas many alternatives use more traditional methods such as silicon strip detectors. Proton beam imaging is a technique that has the potential to replace or augment X-ray imaging, because protons have superior sensitivity to tissue density in the patient compared to X-rays (Koehler, 1968, Depauw and Seco, 2011) and therefore allow for a more precise treatment plan when proton beam therapy is used (Schippers and Lomax, 2011, Penfold et al., 2009, Rietzel et al., 2007, Williams, 2004). One of the two main reasons is that X-ray CT in practice involves a model dependent analysis, wheras proton imaging allows us to directly probe the proton-tissue interaction, omitting inherent imprecisions of the X-ray model. The other reason is that X-ray absorbtion decays exponentially as it traverses matter, whereas protons have a more or less linear absorption. The expensive nature of facilities capable of proton therapy and imaging makes that the techniques are not as common and developed as other methods, but the method is certainly gaining in popularity (Schardt and Elsässer, 2010, Rossi, 2011, Benedikt and Wrulich, 2011, Amaldi and Braccini, 2011).
2.1. Structure of this document¶
This document is laid out as follows: in chapter 3 the context for proton beam imaging will be set. First, it will be illustrated how physics research has a long history of spilling over into medicine and why the application of hadrons (e.g. protons) are now upcoming. Secondly, a short recapitulation of the physics behind hadron imaging and therapy will be presented. Lastly, it will be explained where exactly hadron imaging fits in the broader world of medical imaging methods.
Chapter 4 will discuss current approaches to hadron imaging and will conclude with the Nikhef proposal for a proton beam imager. In chapter 5 the setup will be explained in full detail, as well as the experimental results attained thus far. Chapter 6 will summarize the accomplishments of the Nikhef setup, highlight the points of improvement and discuss the future of the project within the context of hadrons in medicine.